Federico Ramallo
Jul 17, 2024
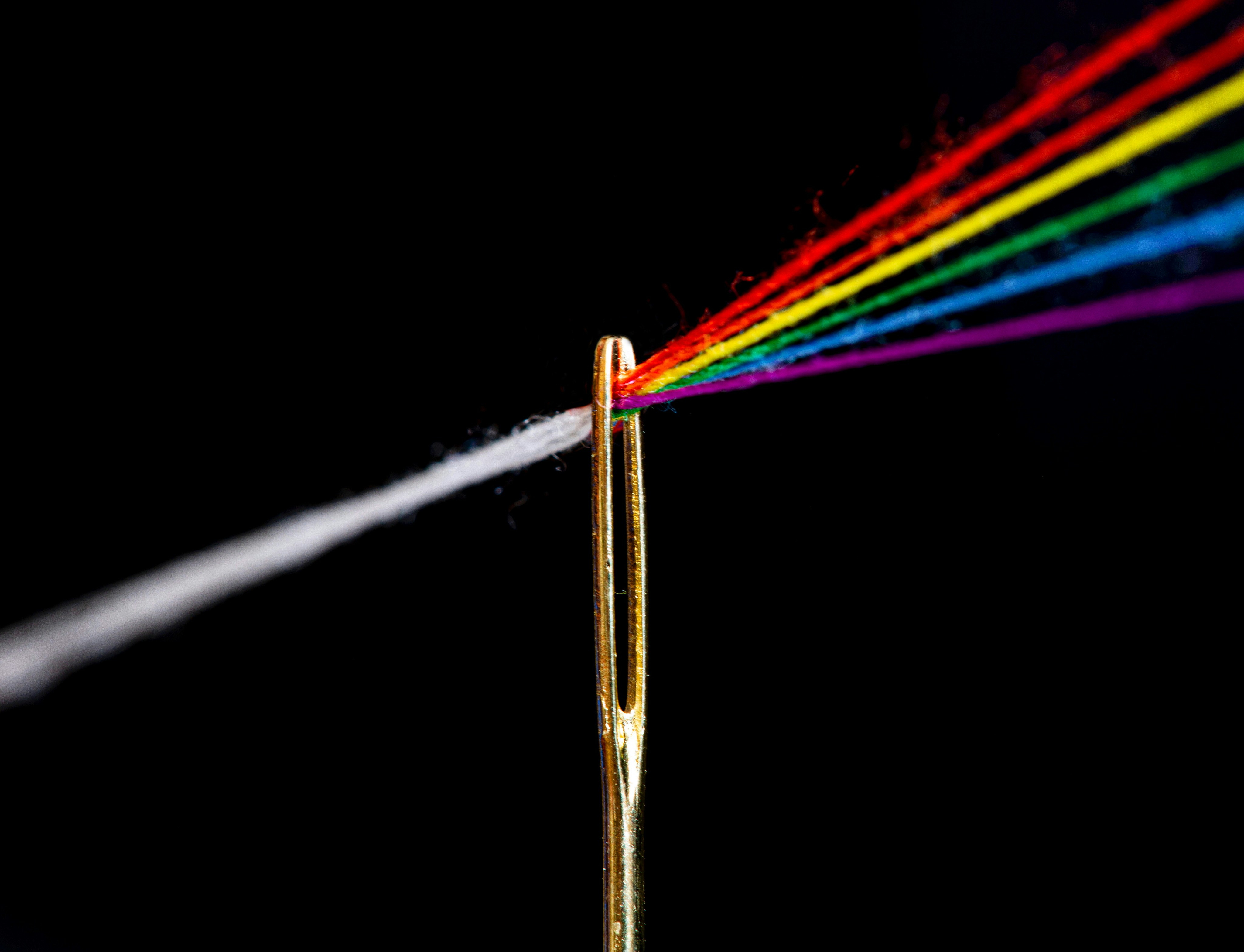
What are LASERS?
Federico Ramallo
Jul 17, 2024
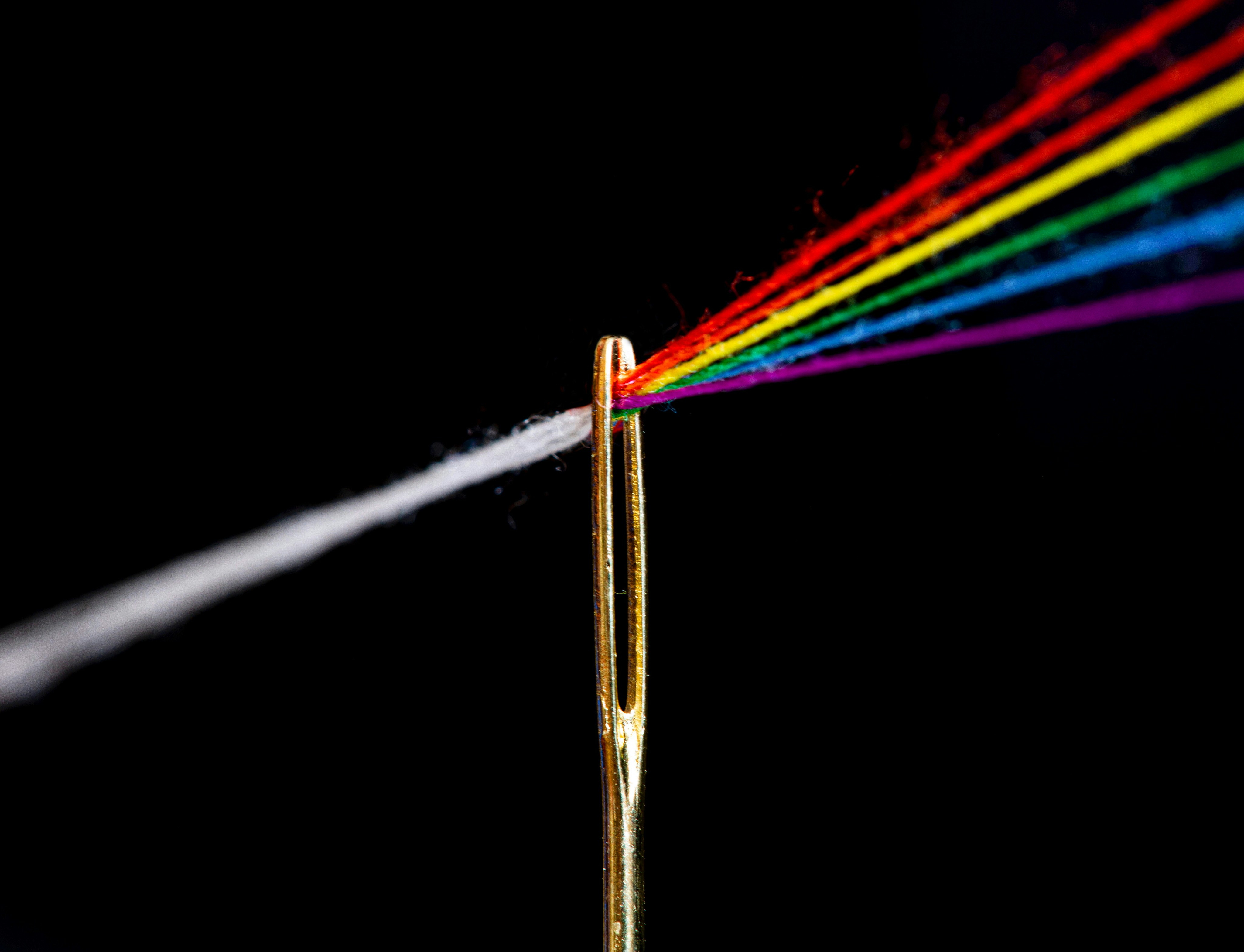
What are LASERS?
Federico Ramallo
Jul 17, 2024
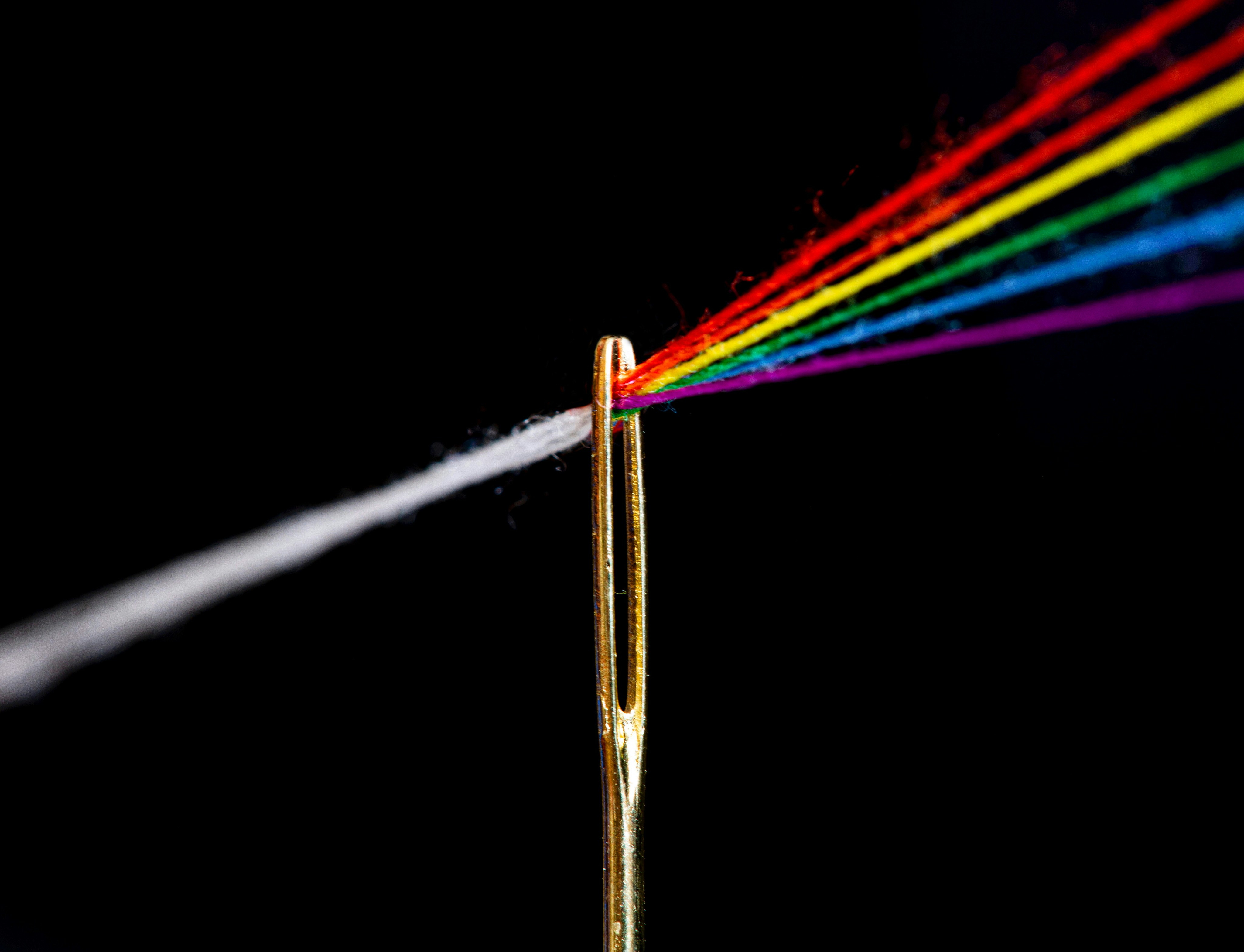
What are LASERS?
Federico Ramallo
Jul 17, 2024
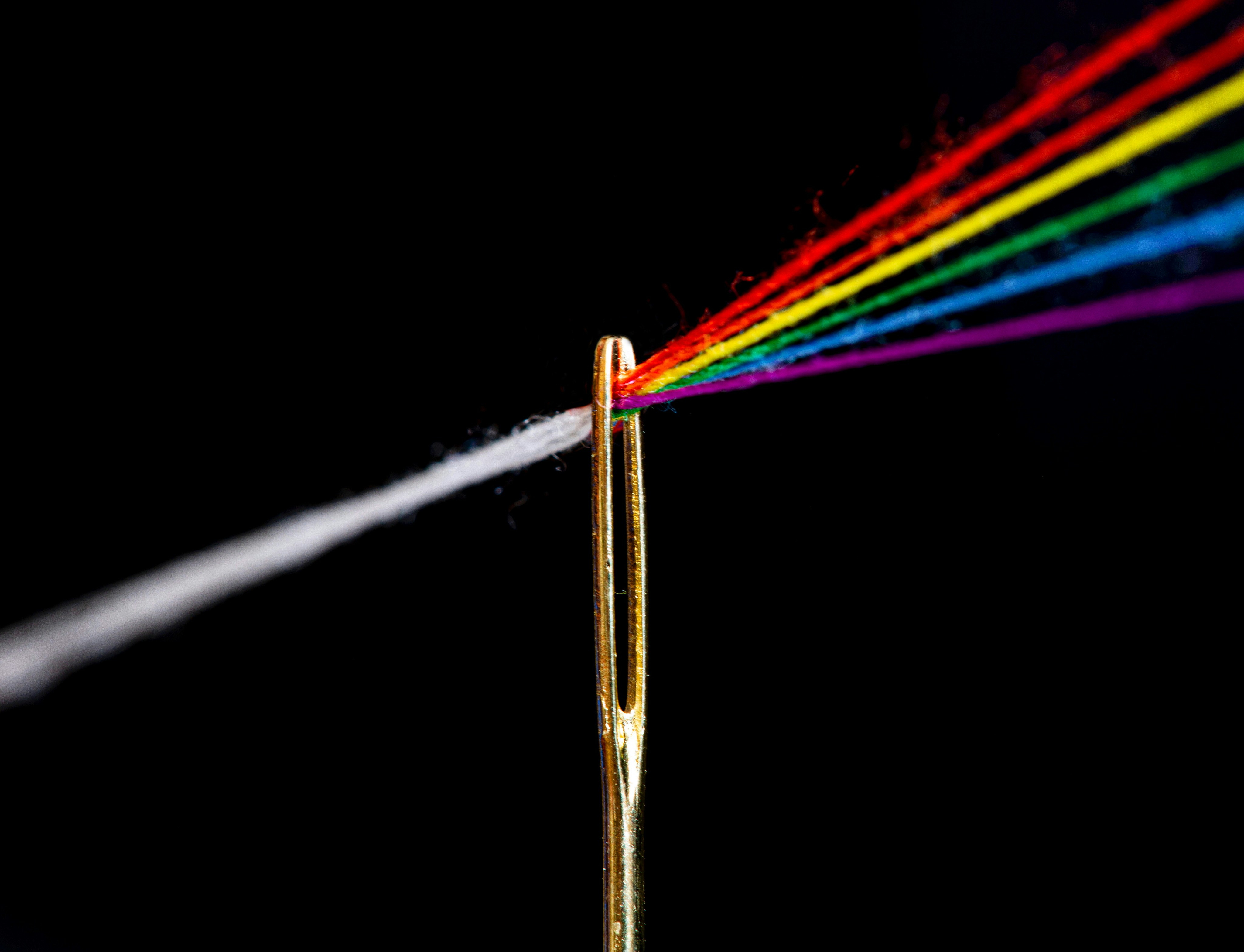
What are LASERS?
Federico Ramallo
Jul 17, 2024
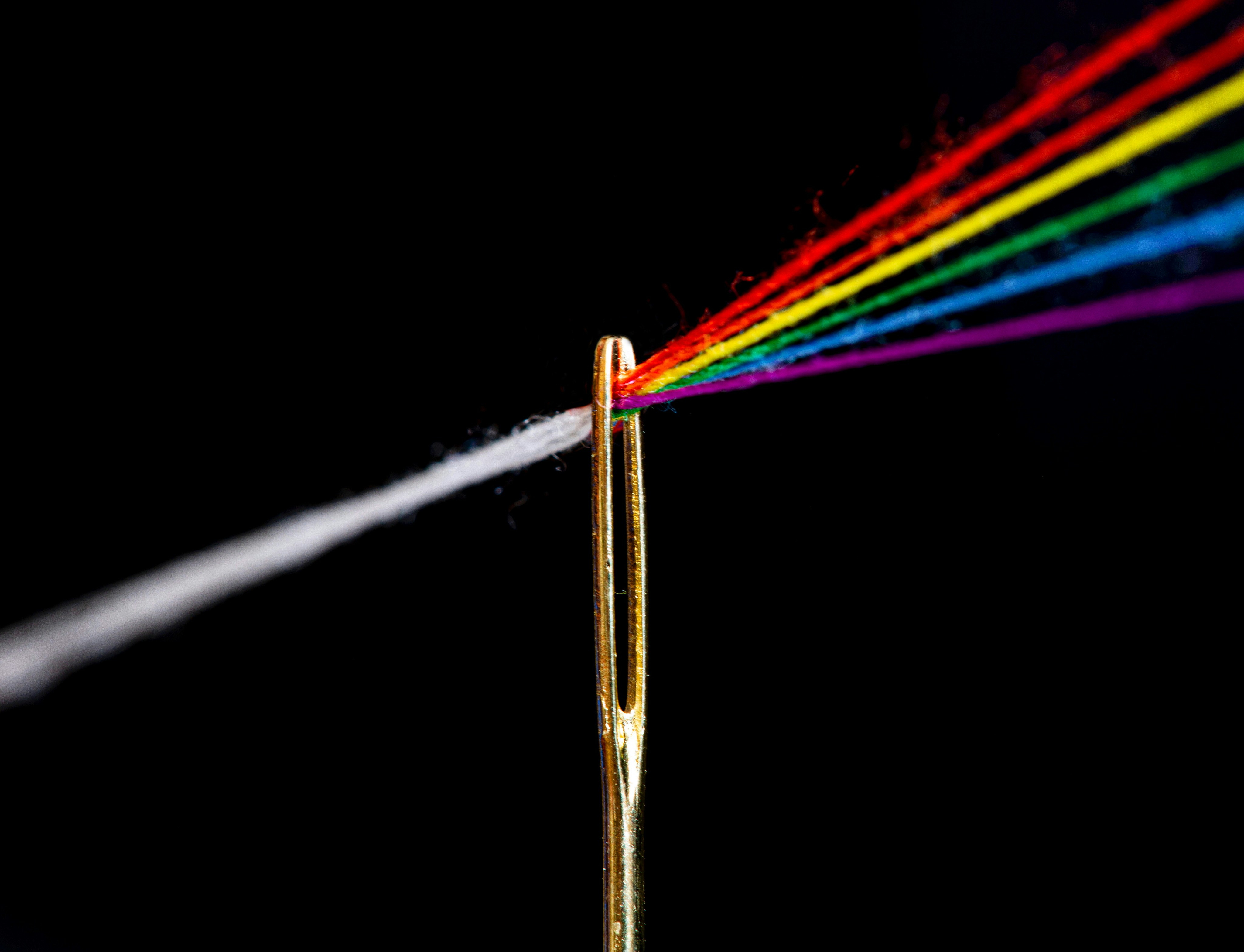
What are LASERS?
Lasers, an abbreviation for Light Amplification by Stimulated Emission of Radiation, represent a fascinating field of physics and engineering that encompasses the production and application of coherent light.
At its core, a laser operates by stimulating the emission of photons from an active medium (such as gases, solids, or liquids) configured within an optical cavity.
This process amplifies light into a powerful, narrow beam that can be finely controlled and directed.
The foundational concept of lasers hinges on three primary properties: directionality, monochromaticity, and coherence. Directionality refers to the laser's ability to emit light in a highly focused and specific direction, as opposed to traditional light sources like bulbs that disperse light in many directions.
This makes lasers incredibly effective for tasks where precision and reach are crucial, such as in surgical procedures or cutting materials.
Monochromaticity is the characteristic of laser light to consist of one color, or wavelength, which is determined by the type of active medium used.
This quality is essential for applications requiring highly specific wavelength, such as in various scientific instruments and fiber optic communications, where a particular wavelength is needed to achieve efficient data transmission.
Coherence, perhaps the most significant property of laser light, involves the light waves being in phase both spatially and temporally.
Spatial coherence allows the light to be focused to a tight spot, and temporal coherence means that the phase of the light waves is consistent over time, which allows for the light to be combined and manipulated in ways that are useful for applications like holography.
The active medium plays a pivotal role in the characteristics of the laser produced. It can be made of various materials, including gases, such as helium-neon, or solids, like ruby or various crystals doped with rare earth elements.
The choice of medium influences the laser's wavelength, power output, and efficiency.
For instance, semiconductor lasers are compact and suitable for electronic applications like CD players and laser printers, while solid-state lasers are used in more demanding applications, including materials processing and range-finding.
Modern lasers are engineered through sophisticated design and precise control over the optical components, including mirrors and resonators that form the cavity.
One mirror is typically highly reflective, while the other allows some light to escape, which is the laser light we use.
The quality of these mirrors and the exact alignment of the components critically affect the efficiency and quality of the laser beam.
High-power lasers, a category that has seen significant technological advances, are specially designed to handle tasks that require intense energy, such as cutting and welding materials in industrial settings or removing tumors in medical procedures.
These lasers need robust cooling systems to manage heat and are typically larger due to the power and infrastructure required.
Moreover, the development of laser technology has continually opened up new applications and improvements in various fields. In telecommunications, lasers transmit vast amounts of data over fiber optic cables.
In medicine, they perform precise surgical cuts and destroy harmful tissues with minimal damage to surrounding areas.
In manufacturing, lasers cut, weld, and polish materials with high precision.
As laser technology progresses, the potential for future innovations continues to expand, promising new advancements in areas such as quantum computing, environmental monitoring, and even space exploration.
The ability of lasers to provide focused energy in a controlled manner makes them a pivotal tool across a wide spectrum of sciences, impacting not just current applications but also paving the way for future technological breakthroughs.
What future innovations in laser technology could significantly impact other fields of science and industry?
Lasers, an abbreviation for Light Amplification by Stimulated Emission of Radiation, represent a fascinating field of physics and engineering that encompasses the production and application of coherent light.
At its core, a laser operates by stimulating the emission of photons from an active medium (such as gases, solids, or liquids) configured within an optical cavity.
This process amplifies light into a powerful, narrow beam that can be finely controlled and directed.
The foundational concept of lasers hinges on three primary properties: directionality, monochromaticity, and coherence. Directionality refers to the laser's ability to emit light in a highly focused and specific direction, as opposed to traditional light sources like bulbs that disperse light in many directions.
This makes lasers incredibly effective for tasks where precision and reach are crucial, such as in surgical procedures or cutting materials.
Monochromaticity is the characteristic of laser light to consist of one color, or wavelength, which is determined by the type of active medium used.
This quality is essential for applications requiring highly specific wavelength, such as in various scientific instruments and fiber optic communications, where a particular wavelength is needed to achieve efficient data transmission.
Coherence, perhaps the most significant property of laser light, involves the light waves being in phase both spatially and temporally.
Spatial coherence allows the light to be focused to a tight spot, and temporal coherence means that the phase of the light waves is consistent over time, which allows for the light to be combined and manipulated in ways that are useful for applications like holography.
The active medium plays a pivotal role in the characteristics of the laser produced. It can be made of various materials, including gases, such as helium-neon, or solids, like ruby or various crystals doped with rare earth elements.
The choice of medium influences the laser's wavelength, power output, and efficiency.
For instance, semiconductor lasers are compact and suitable for electronic applications like CD players and laser printers, while solid-state lasers are used in more demanding applications, including materials processing and range-finding.
Modern lasers are engineered through sophisticated design and precise control over the optical components, including mirrors and resonators that form the cavity.
One mirror is typically highly reflective, while the other allows some light to escape, which is the laser light we use.
The quality of these mirrors and the exact alignment of the components critically affect the efficiency and quality of the laser beam.
High-power lasers, a category that has seen significant technological advances, are specially designed to handle tasks that require intense energy, such as cutting and welding materials in industrial settings or removing tumors in medical procedures.
These lasers need robust cooling systems to manage heat and are typically larger due to the power and infrastructure required.
Moreover, the development of laser technology has continually opened up new applications and improvements in various fields. In telecommunications, lasers transmit vast amounts of data over fiber optic cables.
In medicine, they perform precise surgical cuts and destroy harmful tissues with minimal damage to surrounding areas.
In manufacturing, lasers cut, weld, and polish materials with high precision.
As laser technology progresses, the potential for future innovations continues to expand, promising new advancements in areas such as quantum computing, environmental monitoring, and even space exploration.
The ability of lasers to provide focused energy in a controlled manner makes them a pivotal tool across a wide spectrum of sciences, impacting not just current applications but also paving the way for future technological breakthroughs.
What future innovations in laser technology could significantly impact other fields of science and industry?
Lasers, an abbreviation for Light Amplification by Stimulated Emission of Radiation, represent a fascinating field of physics and engineering that encompasses the production and application of coherent light.
At its core, a laser operates by stimulating the emission of photons from an active medium (such as gases, solids, or liquids) configured within an optical cavity.
This process amplifies light into a powerful, narrow beam that can be finely controlled and directed.
The foundational concept of lasers hinges on three primary properties: directionality, monochromaticity, and coherence. Directionality refers to the laser's ability to emit light in a highly focused and specific direction, as opposed to traditional light sources like bulbs that disperse light in many directions.
This makes lasers incredibly effective for tasks where precision and reach are crucial, such as in surgical procedures or cutting materials.
Monochromaticity is the characteristic of laser light to consist of one color, or wavelength, which is determined by the type of active medium used.
This quality is essential for applications requiring highly specific wavelength, such as in various scientific instruments and fiber optic communications, where a particular wavelength is needed to achieve efficient data transmission.
Coherence, perhaps the most significant property of laser light, involves the light waves being in phase both spatially and temporally.
Spatial coherence allows the light to be focused to a tight spot, and temporal coherence means that the phase of the light waves is consistent over time, which allows for the light to be combined and manipulated in ways that are useful for applications like holography.
The active medium plays a pivotal role in the characteristics of the laser produced. It can be made of various materials, including gases, such as helium-neon, or solids, like ruby or various crystals doped with rare earth elements.
The choice of medium influences the laser's wavelength, power output, and efficiency.
For instance, semiconductor lasers are compact and suitable for electronic applications like CD players and laser printers, while solid-state lasers are used in more demanding applications, including materials processing and range-finding.
Modern lasers are engineered through sophisticated design and precise control over the optical components, including mirrors and resonators that form the cavity.
One mirror is typically highly reflective, while the other allows some light to escape, which is the laser light we use.
The quality of these mirrors and the exact alignment of the components critically affect the efficiency and quality of the laser beam.
High-power lasers, a category that has seen significant technological advances, are specially designed to handle tasks that require intense energy, such as cutting and welding materials in industrial settings or removing tumors in medical procedures.
These lasers need robust cooling systems to manage heat and are typically larger due to the power and infrastructure required.
Moreover, the development of laser technology has continually opened up new applications and improvements in various fields. In telecommunications, lasers transmit vast amounts of data over fiber optic cables.
In medicine, they perform precise surgical cuts and destroy harmful tissues with minimal damage to surrounding areas.
In manufacturing, lasers cut, weld, and polish materials with high precision.
As laser technology progresses, the potential for future innovations continues to expand, promising new advancements in areas such as quantum computing, environmental monitoring, and even space exploration.
The ability of lasers to provide focused energy in a controlled manner makes them a pivotal tool across a wide spectrum of sciences, impacting not just current applications but also paving the way for future technological breakthroughs.
What future innovations in laser technology could significantly impact other fields of science and industry?
Lasers, an abbreviation for Light Amplification by Stimulated Emission of Radiation, represent a fascinating field of physics and engineering that encompasses the production and application of coherent light.
At its core, a laser operates by stimulating the emission of photons from an active medium (such as gases, solids, or liquids) configured within an optical cavity.
This process amplifies light into a powerful, narrow beam that can be finely controlled and directed.
The foundational concept of lasers hinges on three primary properties: directionality, monochromaticity, and coherence. Directionality refers to the laser's ability to emit light in a highly focused and specific direction, as opposed to traditional light sources like bulbs that disperse light in many directions.
This makes lasers incredibly effective for tasks where precision and reach are crucial, such as in surgical procedures or cutting materials.
Monochromaticity is the characteristic of laser light to consist of one color, or wavelength, which is determined by the type of active medium used.
This quality is essential for applications requiring highly specific wavelength, such as in various scientific instruments and fiber optic communications, where a particular wavelength is needed to achieve efficient data transmission.
Coherence, perhaps the most significant property of laser light, involves the light waves being in phase both spatially and temporally.
Spatial coherence allows the light to be focused to a tight spot, and temporal coherence means that the phase of the light waves is consistent over time, which allows for the light to be combined and manipulated in ways that are useful for applications like holography.
The active medium plays a pivotal role in the characteristics of the laser produced. It can be made of various materials, including gases, such as helium-neon, or solids, like ruby or various crystals doped with rare earth elements.
The choice of medium influences the laser's wavelength, power output, and efficiency.
For instance, semiconductor lasers are compact and suitable for electronic applications like CD players and laser printers, while solid-state lasers are used in more demanding applications, including materials processing and range-finding.
Modern lasers are engineered through sophisticated design and precise control over the optical components, including mirrors and resonators that form the cavity.
One mirror is typically highly reflective, while the other allows some light to escape, which is the laser light we use.
The quality of these mirrors and the exact alignment of the components critically affect the efficiency and quality of the laser beam.
High-power lasers, a category that has seen significant technological advances, are specially designed to handle tasks that require intense energy, such as cutting and welding materials in industrial settings or removing tumors in medical procedures.
These lasers need robust cooling systems to manage heat and are typically larger due to the power and infrastructure required.
Moreover, the development of laser technology has continually opened up new applications and improvements in various fields. In telecommunications, lasers transmit vast amounts of data over fiber optic cables.
In medicine, they perform precise surgical cuts and destroy harmful tissues with minimal damage to surrounding areas.
In manufacturing, lasers cut, weld, and polish materials with high precision.
As laser technology progresses, the potential for future innovations continues to expand, promising new advancements in areas such as quantum computing, environmental monitoring, and even space exploration.
The ability of lasers to provide focused energy in a controlled manner makes them a pivotal tool across a wide spectrum of sciences, impacting not just current applications but also paving the way for future technological breakthroughs.
What future innovations in laser technology could significantly impact other fields of science and industry?
Lasers, an abbreviation for Light Amplification by Stimulated Emission of Radiation, represent a fascinating field of physics and engineering that encompasses the production and application of coherent light.
At its core, a laser operates by stimulating the emission of photons from an active medium (such as gases, solids, or liquids) configured within an optical cavity.
This process amplifies light into a powerful, narrow beam that can be finely controlled and directed.
The foundational concept of lasers hinges on three primary properties: directionality, monochromaticity, and coherence. Directionality refers to the laser's ability to emit light in a highly focused and specific direction, as opposed to traditional light sources like bulbs that disperse light in many directions.
This makes lasers incredibly effective for tasks where precision and reach are crucial, such as in surgical procedures or cutting materials.
Monochromaticity is the characteristic of laser light to consist of one color, or wavelength, which is determined by the type of active medium used.
This quality is essential for applications requiring highly specific wavelength, such as in various scientific instruments and fiber optic communications, where a particular wavelength is needed to achieve efficient data transmission.
Coherence, perhaps the most significant property of laser light, involves the light waves being in phase both spatially and temporally.
Spatial coherence allows the light to be focused to a tight spot, and temporal coherence means that the phase of the light waves is consistent over time, which allows for the light to be combined and manipulated in ways that are useful for applications like holography.
The active medium plays a pivotal role in the characteristics of the laser produced. It can be made of various materials, including gases, such as helium-neon, or solids, like ruby or various crystals doped with rare earth elements.
The choice of medium influences the laser's wavelength, power output, and efficiency.
For instance, semiconductor lasers are compact and suitable for electronic applications like CD players and laser printers, while solid-state lasers are used in more demanding applications, including materials processing and range-finding.
Modern lasers are engineered through sophisticated design and precise control over the optical components, including mirrors and resonators that form the cavity.
One mirror is typically highly reflective, while the other allows some light to escape, which is the laser light we use.
The quality of these mirrors and the exact alignment of the components critically affect the efficiency and quality of the laser beam.
High-power lasers, a category that has seen significant technological advances, are specially designed to handle tasks that require intense energy, such as cutting and welding materials in industrial settings or removing tumors in medical procedures.
These lasers need robust cooling systems to manage heat and are typically larger due to the power and infrastructure required.
Moreover, the development of laser technology has continually opened up new applications and improvements in various fields. In telecommunications, lasers transmit vast amounts of data over fiber optic cables.
In medicine, they perform precise surgical cuts and destroy harmful tissues with minimal damage to surrounding areas.
In manufacturing, lasers cut, weld, and polish materials with high precision.
As laser technology progresses, the potential for future innovations continues to expand, promising new advancements in areas such as quantum computing, environmental monitoring, and even space exploration.
The ability of lasers to provide focused energy in a controlled manner makes them a pivotal tool across a wide spectrum of sciences, impacting not just current applications but also paving the way for future technological breakthroughs.
What future innovations in laser technology could significantly impact other fields of science and industry?
Guadalajara
Werkshop - Av. Acueducto 6050, Lomas del bosque, Plaza Acueducto. 45116,
Zapopan, Jalisco. México.
Texas
5700 Granite Parkway, Suite 200, Plano, Texas 75024.
© Density Labs. All Right reserved. Privacy policy and Terms of Use.
Guadalajara
Werkshop - Av. Acueducto 6050, Lomas del bosque, Plaza Acueducto. 45116,
Zapopan, Jalisco. México.
Texas
5700 Granite Parkway, Suite 200, Plano, Texas 75024.
© Density Labs. All Right reserved. Privacy policy and Terms of Use.
Guadalajara
Werkshop - Av. Acueducto 6050, Lomas del bosque, Plaza Acueducto. 45116,
Zapopan, Jalisco. México.
Texas
5700 Granite Parkway, Suite 200, Plano, Texas 75024.
© Density Labs. All Right reserved. Privacy policy and Terms of Use.